Research
当研究室は、合成生物学を拡張することで、次世代の産業を生み出す革新的バイオテクノロジーの創出に挑戦しています。
By expanding synthetic biology, our lab is taking on the challenge of creating innovative biotechnology that will build the next generation of industry..
遺伝暗号拡張による次世代産業の創出
Creating the next generation of industries by expanding the genetic code
ペプチド・タンパク質の再発明
Reinventing peptides and proteins
生命が40億年かけて進化させてきたペプチド・タンパク質は、その多様な機能性から、さまざまな産業で活用されています。例えば食品分野や化学分野では、物質生産のために多様な酵素が利用されています。また製薬分野では、高い安全性と効果を併せ持つペプチド医薬や抗体医薬が活躍しています。
しかし、人類が活用できるペプチド・タンパク質の多様性には、実は大きな制約が存在します。なぜなら、現生生命は、ペプチド・タンパク質を合成するためにたった20種類の天然アミノ酸しか使用できないからです。
Peptides and proteins, which have evolved over four billion years of life, are used in a wide range of industries due to their diverse functionality. In the food and chemical industries, for example, a wide range of enzymes are used to produce substances. The pharmaceutical industry uses peptide and antibody drugs with high safety and efficacy.
However, the variety of peptides and proteins that can be used by humans is limited. This is because living organisms can only use 20 types of canonical amino acids to synthesize peptides and proteins.
当研究室では、遺伝暗号を拡張し、20種を遥かに超えるモノマーを自由自在に重合可能とすることで、より高い安定性・特異性・活性を持つ次世代ペプチド・タンパク質の創出に挑戦しています。
遺伝暗号拡張に向けた当研究室のコア技術のひとつが、リボソームエンジニアリングです。リボソームとは、遺伝コードに従って20種類の天然アミノ酸を重合する超分子です。リボソームの触媒活性を改変できれば多様な非天然モノマーを重合可能になると期待されますが、リボソームは生命の必須因子であり、その触媒活性を改変することは難しい課題でした。当研究室では、試験管内でリボソームを合成することに世界で初めて成功し、細胞毒性を考慮することなく自由自在にリボソームを改変することを可能としました。
In our laboratory, we are working to create next-generation peptides and proteins with greater stability, specificity, and activity by extending the genetic code to allow polymerization of monomers far beyond the 20 canonical amino acids.
One of our core technologies for extending the genetic code is ribosome engineering. Ribosomes are supramolecular complexes that polymerize the 20 canonical amino acids according to the genetic code. If the catalytic activity of ribosomes could be modified, it would be possible to polymerize a wide variety of unnatural monomers, but ribosomes are essential factors of life, and modifying their catalytic activity has been a difficult challenge. In our laboratory, we were the first in the world to synthesize ribosomes in the test tube, making it possible to freely modify ribosomes without having to consider their cytotoxicity.
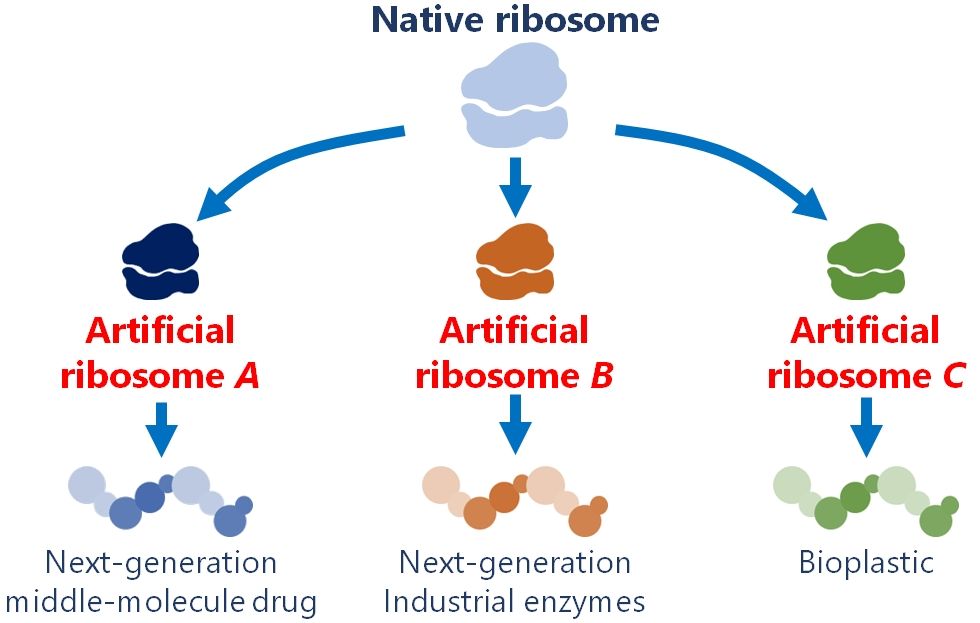
私達はリボソームエンジニアリングにより、人類が利用可能なペプチド・タンパク質の拡張に挑戦しています。当研究室が目指すゴールのひとつが、D-アミノ酸を効率的に重合可能な人工リボソームを創出し、「鏡像タンパク質工学」を実現することです。D-アミノ酸から成るD-タンパク質は、L-タンパク質が触媒する反応のキラリティを反転できるため、人類が利用可能な化合物空間を倍に広げられます。また、D-タンパク質はプロテアーゼ耐性が高く分解されにくいため、次世代の産業用酵素や消化管へ投与可能なタンパク質医薬として活躍すると期待されます。
We are currently working to expand the range of peptides and proteins that can be used by humans through ribosome engineering. One of the goals of our laboratory is to create an artificial ribosome that can efficiently polymerize D-amino acids and realize “mirror-image protein engineering". D-proteins, which are composed of D-amino acids, can reverse the chirality of reactions catalyzed by L-proteins, thus doubling the range of compounds that can be used by humans. In addition, D-proteins are highly resistant to proteases and difficult to degrade, so they are expected to play an active role as next-generation industrial enzymes and protein pharmaceuticals that can be administered through the digestive tract.
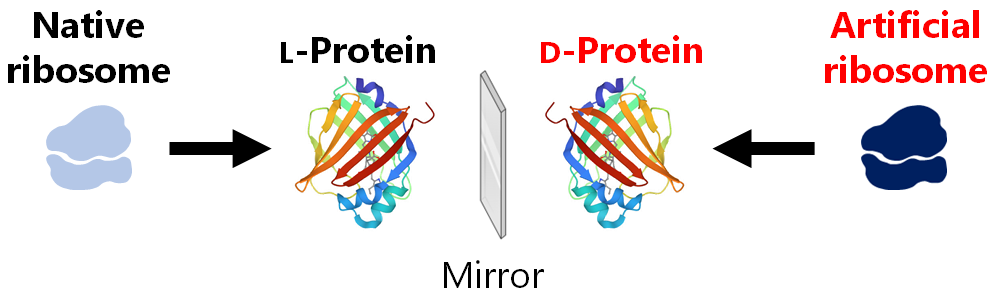
それ以外にも例えば、膜透過性が高い非天然アミノ酸を重合できるようになれば、細胞内の病原因子を攻撃できる次世代医薬の創出に繋がるでしょう。また、共有結合を形成し得る高い反応性の非天然アミノ酸を重合できるようになれば、不可逆的にターゲットに結合して高い薬効を示す共有結合型抗体医薬の創出に繋がります。さらに、ペプチド結合ではなくエステル結合を効率的に重合できるようになれば、既存のペプチド・タンパク質とは根本的に性質が異なる多様なバイオポリマーの合成が可能になると期待されます。
In addition, if it becomes possible, for example, to polymerize unnatural amino acids with high membrane permeability, this will lead to the creation of next-generation pharmaceuticals that can attack pathogenic factors inside cells. Furthermore, if it becomes possible to polymerize highly reactive unnatural amino acids that can form covalent bonds, this will lead to the creation of covalent antibody drugs that bind irreversibly to targets and exhibit high efficacy. In addition, if it becomes possible to efficiently polymerize ester bonds rather than peptide bonds, it is expected that it will become possible to synthesize a wide variety of biopolymers with properties fundamentally different from existing peptides and proteins.
生命のリデザイン
Redesigning life
現代の細胞工学は、現生生命を足場として利用しています。それにより、2つの根本的制約が存在します。第一に、現生生命には多数のブラックボックスが存在することです。第二に、現生生命のゲノムのブループリントに従わなければならないことです。そのため、生命を自在に操作して自然を超越する機能を創出することは、いまだ難しい課題です。
Modern cell engineering uses living organisms as a chassis. This has two limitations. First, there are many black boxes in the living organism. Second, we have to follow the blueprint of their genome. Therefore, it is still a difficult task to manipulate life at will and create functions that go beyond nature.
現代の細胞工学の制約を乗り越え、自然を超越する生命機能を自由にデザイン可能とするためには,自己複製能のみを持つ最小の人工細胞を構築し、「進化人工細胞工学」と呼べる新たな学問領域を創出することが重要です。そのような人工細胞を構築できれば、デザインされた遺伝子・遺伝子ネットワーク・ゲノムを付与することで、物理法則が許す範囲であらゆる表現型を自由に現出させられるようになるでしょう。
最小の人工細胞を創出するためには、生命の最小のオペレーティングシステム「自律的セントラルドグマ」を再構成する必要があります。自律的セントラルドグマとは、転写・翻訳・DNA複製・リボソーム自己複製という4つのプロセスを、自らにコードされる情報に基づいて実行し、自己を複製し続けるプロセスです。自律的セントラルドグマを構成する要素のうち、転写・翻訳・DNA複製の再構成は60年前に実現されていましたが、リボソーム自己複製の再構成は極めて難しく、誰も実現に成功しておりませんでした。私たちは近年、世界で初めてリボソーム自己複製を試験管内で起動することに成功し、人工生命の創出に向け大きなブレークスルーを実現しています。
In order to overcome the limitations of modern cell engineering and make it possible to freely design biological functions that go beyond nature, it is important to construct the simplest artificial cell with only the ability to self-replicate and create a new field of research called "evolutionary artificial cell engineering". If we can construct such artificial cells, we will be able to give them designer genes, gene networks, and genomes to freely manifest any phenotype as far as the laws of physics allow.
To create the simplest artificial cell, it is necessary to reconstitute the simplest operating system of life, the autonomous central dogma. Autonomous central dogma is a process that carries out four processes - transcription, translation, DNA replication, and ribosome self-replication - based on the information encoded in itself, and continues to replicate itself. Among the four processes of the autonomous central dogma, the reconstitution of transcription, translation, and DNA replication had been realized 60 years ago, but the reconstitution of ribosome self-replication was extremely difficult, and no one had succeeded. Recently, we have successfully reconstructed ribosome self-replication in vitro, achieving a major breakthrough in the creation of artificial life.
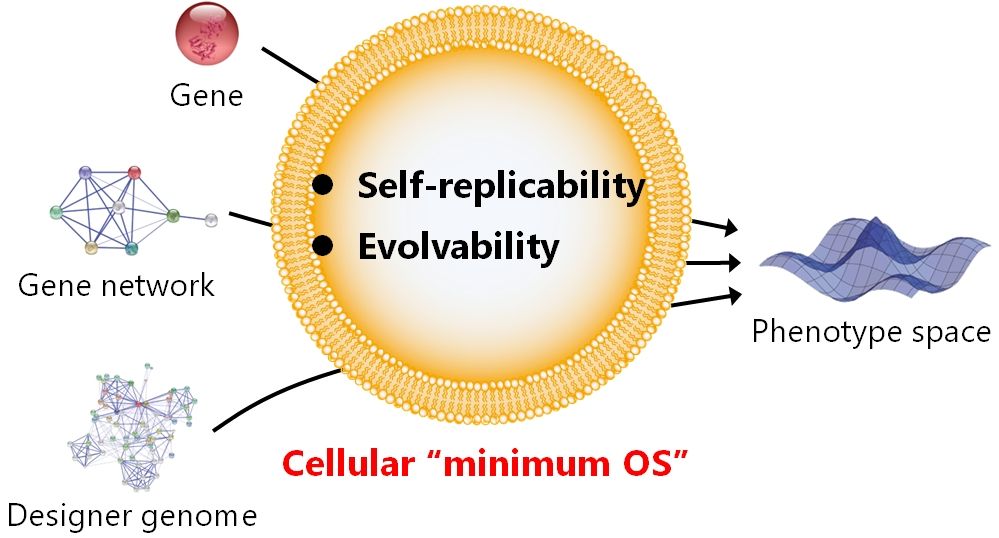
生命機能をシステムレベルで理解する
Understanding biological functions at the system level
革新的バイオテクノロジーは生命の深い理解から生まれます。そこで私たちは、これまで測れなかったものを測定可能とする次世代オミックス技術(網羅的分析技術)を開発し、それをさまざまな生命現象に適用することで、生命をより深く・総合的に理解しようと試みています。
Innovative biotechnology comes from a deep understanding of life. Therefore, we are developing next-generation omics technologies that can measure things that were previously unmeasurable, and applying these technologies to various biological phenomena to gain a deeper and more comprehensive understanding of life.
次世代オミックスによる生命現象のデータ駆動型解析
Data-driven analysis of biological phenomena through next-generation omics
オミックス解析とは、生命現象を包括的に理解するための方法論です。オミックス解析は、生命における各階層(DNA・RNA・タンパク質・代謝物・細胞)の網羅的解析を実現することで、生命現象の理解や病気のメカニズム解明などに大きく貢献しています。しかしながら、現在のオミックス解析は感度・網羅性に課題を抱えており、あらゆる生体因子を網羅的に分析可能にはなっていません。
当研究室は、生命現象のより深い理解を実現するために、感度・網羅性に優れた次世代オミックス技術を開発しています。具体例のひとつが、ナノポアを活用した1分子プロテオミクスです。次世代シーケンサーは、DNA・RNAを1分子レベルで網羅的に解析することに成功し、生命科学のあり方を変えました。しかし、生命機能の実行因子である「タンパク質」に対しては、そのような方法論は存在せず、生命科学の大きなボトルネックになっています。当研究室では、ナノポアと呼ばれる、微小な穴を形成するタンパク質を活用することで、タンパク質を1分子レベルで網羅的に解析することに成功しました。また当研究室では、オミックスにより得られた莫大なデータセットに対し、ガウス過程回帰などの機械学習を適用することで、複雑な生命現象を高い精度でモデル化しようと試みています。これらの技術を統合・応用することで、さまざまな生命現象をデータ駆動で理解することが可能になりつつあります。
Omics analysis is a methodology for the comprehensive understanding of biological phenomena. Omics analysis contributes greatly to the understanding of biological phenomena and the elucidation of disease mechanisms by comprehensively analyzing each level of life (DNA, RNA, proteins, metabolites, and cells). However, current omics analysis is limited in sensitivity and comprehensiveness, and it is not yet possible to comprehensively analyze all biological factors.
In order to gain a deeper understanding of biological phenomena, our laboratory is developing next-generation omics technologies that excel in sensitivity and comprehensiveness. A specific example is single molecule proteomics using nanopores. Next-generation sequencing has succeeded in comprehensively analyzing DNA and RNA at the single-molecule level and has transformed the life sciences. However, no such method exists for proteins, which carry out biological functions, and this is a major bottleneck in life sciences. In our lab, we have succeeded in comprehensively analyzing proteins at the single-molecule level by using proteins that form microscopic holes called nanopores. In addition, our lab is attempting to model complex biological phenomena with high accuracy by applying machine learning techniques, such as Gaussian process regression, to the massive data sets generated by omics. By integrating and applying these technologies, it is becoming possible to understand various biological phenomena in a data-driven manner.
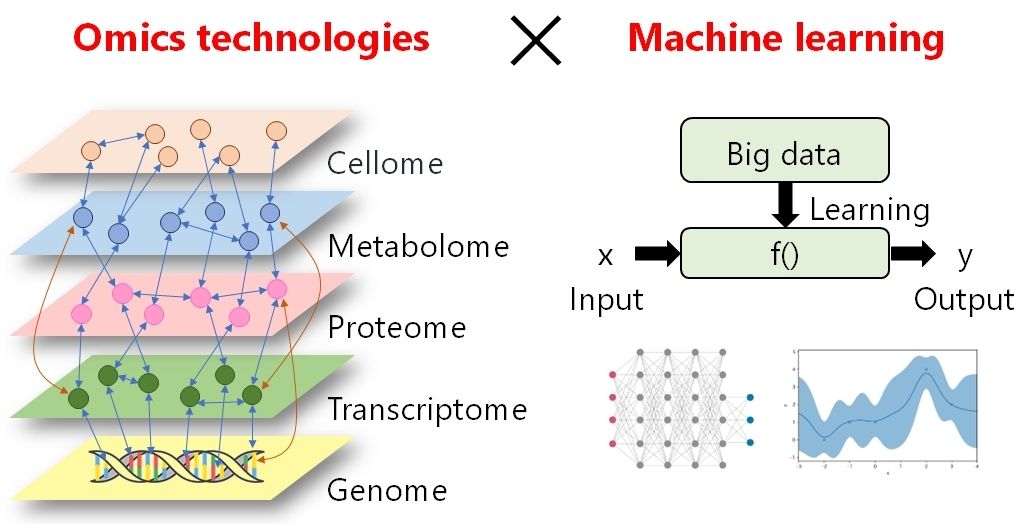
微生物叢のデータ駆動型解析
Data-driven microbiome analysis
微生物は、極めて多様な機能を持つ生物群です。高圧環境・高熱環境・嫌気環境・好気環境など地球上のあらゆる環境に、ユニークな機能を持つ微生物が存在しています。また、動物や植物は、微生物叢と共生する超生命体として存在しており、その生存にとって個体-微生物叢間相互作用は重要な役割を担っています。しかし、このように多様な微生物を網羅的に分析するための方法論は不足しており、いまだ多数のブラックボックスが存在しています。当研究室は、次世代オミックス技術を用いて、微生物叢の機能をシステマティックに解明しようと試みています。
Microorganisms are a group of organisms with extremely diverse functions. Microbes with unique functions exist in every environment on Earth, including high-pressure, hyperthermal, anaerobic, and aerobic environments. In addition, animals and plants exist as superorganisms that live in symbiosis with the microflora, and individual-microflora interactions play an important role in their survival. However, methods for comprehensive analysis of such diverse microorganisms are still lacking, and many black boxes remain. Our laboratory is attempting to systematically elucidate functions of the microflora through next-generation omics technologies.
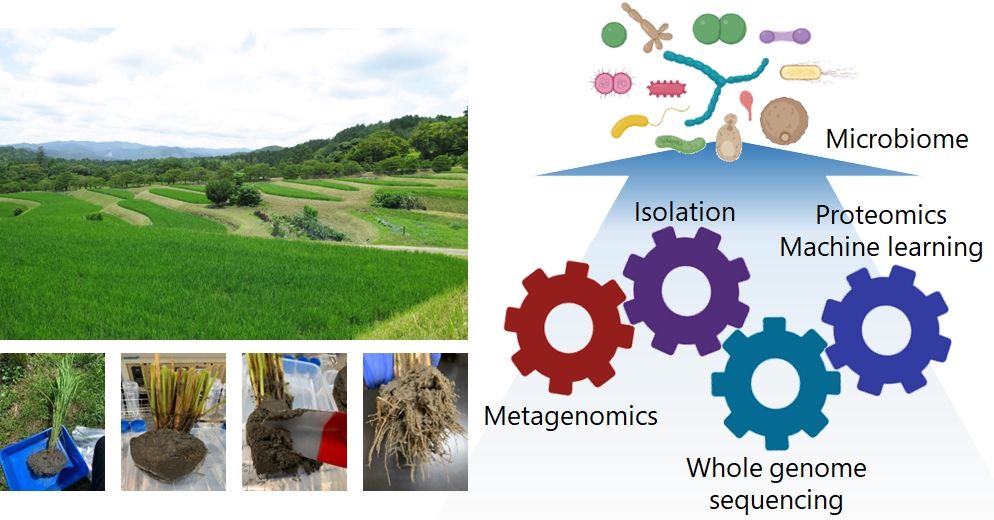
次世代抗体の創出
Creating the next generation of antibodies
重鎖抗体の可変領域であるナノボディは、従来の抗体と比べて同等の親和性を有しつつ、組織浸透性や生産性などに優れ、次世代抗体のひとつとして注目を集めています。
ナノボディの結合力をハイスループットに評価するために、ファージディスプレイや酵母ディスプレイが一般的に用いられます。しかし、ファージや酵母などの担体に固定化したナノボディは遊離状態と異なるプロファイルを示すことも多いという欠点がありました。
そこで私たちは、遊離型のナノボディの特性をハイスループットに評価可能とする新規方法論「ペプチドバーコーディング」を開発しています。この技術に基づいてナノボディ探索を手掛けるバイオベンチャーBarcodebody社を設立しました。現在、アカデミアや企業と多数の共同開発プロジェクトが進んでいます。
Nanobodies, the variable regions of heavy chain antibodies, are emerging as one of the next generation of antibodies due to their superior tissue penetration and productivity while having the same affinity as conventional antibodies.
Phage and yeast displays are commonly used for high-throughput evaluation of nanobody binding ability. However, nanobodies immobilized on carriers such as phage or yeast have the disadvantage that they often exhibit profiles that differ from those in the free state.
Therefore, we are developing a novel methodology, peptide barcoding, which allows high-throughput characterization of free nanobodies. Based on this technology, we have founded Barcodebody, a biotech company dedicated to nanobody discovery. Numerous joint development projects with academia and industry are currently underway.
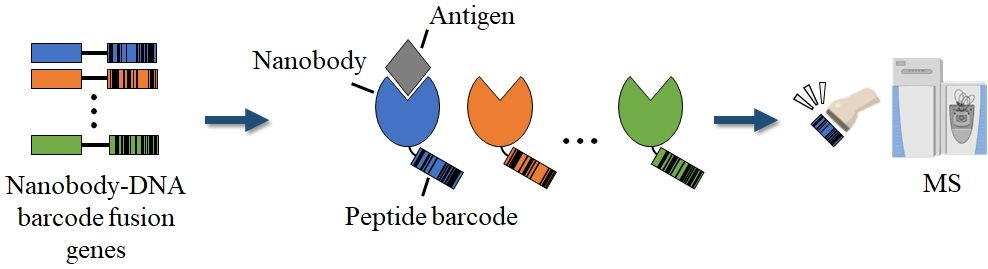
キーワード
オミックス, ゲノム, トランスクリプトーム, プロテオーム, メタボローム, セローム, 情報科学, 合成生物学, データ駆動型サイエンス, 微生物, 微生物叢, 人工細胞, 人工生命, リボソーム, 中分子医薬, 代謝工学, バイオものづくり, ナノボディ, 抗体, ナノポア